Introduction
Welcome to the first of a ten part series on the fundamentals of aircraft design. The aim of this series is to give you an introduction to the principles used by engineers in the design of a new aircraft. We start at the very beginning with Fundamentals of Flight, and progress through the various components of the aircraft: wing, fuselage, tail design. Finally we'll end off with a off with some basic mathematical modelling of aircraft performance.
Aircraft, like birds, are heavier than air. Therefore in order for an aircraft to fly, it is logical that it must overcome the effects of gravity by producing a lifting force greater than or equal to its weight. On a conventional aircraft this lifting force is produced by the wing. We won’t go into too much detail yet (see the post on wing design), suffice to say that the size and shape of the wing is what induces a lifting force that is able to lift the plane into the air.
Four Fundamental Forces
There are four fundamental forces that govern how an aircraft flies and its state of flight at any one time. These four forces are: LIFT, WEIGHT, THRUST & DRAG.
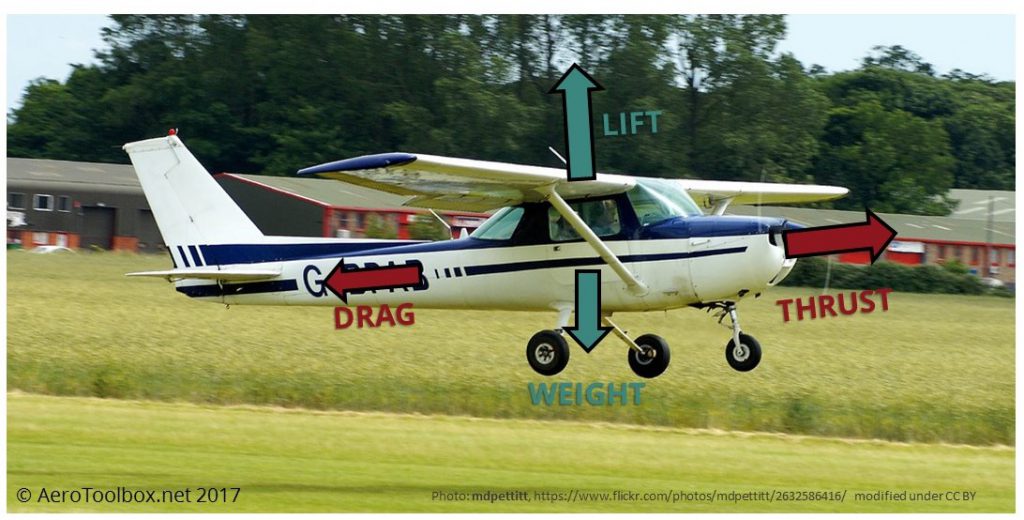
These four forces represent a push or pull in a particular direction. Since force is not only described by a magnitude but also a direction, we define force as a vector quantity.
Take a look at the image above which shows a Cessna 150M taking off from a grass runway. The four fundamental forces and the direction each one of them is acting is superimposed onto the image. In the take-off case, the aircraft is accelerating forward such that the thrust produced by the engine is greater than the drag resisting the aircraft motion. During the initial stages of take-off, aircraft is accelerating upward away from the runway, and the net upward force must be greater than the aircraft weight.
Let’s go through each of the four forces in a little more detail:
Weight
Weight is a force that is developed as a by-product of the mass of the aircraft. All matter on earth has a mass, and the weight of the aircraft is the gravitational pull of the earth acting on the aircraft's mass. The total aircraft mass comprises of structure, engines, payload (people, luggage, freight), fuel, systems, and anything else that is on board at the time of flight. The total weight of the aircraft will vary during flight as fuel is consumed by the engines but we assume the mass is constant for a given snap-shot in time. The mass of the aircraft is distributed throughout it's volume but often times the total mass of the airplane is lumped into a single point called the center of mass. This can be thought of as the location at which the aircraft would remain balanced if suspended from that point. The total weight of the aircraft can be represented by a force vector (weight) acting at the center of gravity (corresponding to the center of mass). An aircraft has to be designed to operate safely within a range of center of gravity positions, thus the control surfaces have to be designed to balance or trim the aircraft throughout this range.
The total weight of the aircraft is represented at the center of gravity and is a vector that is always directed downward toward the center of the earth.
Lift
In order to counteract the weight force, an aircraft must produce a lifting force. This lifting force is generated by the motion of air flowing over the airplane. The majority of the lifting force is produced by the aircraft’s wing. The shape or curvature of the wing section is very important as this is what produces a pressure variation between the upper and lower wing surface which in turn induces this lifting force. We will go into far more detail as to how the wing section produces lift in a later post and you will also learn how the size and dimensions of the wing have a large effect in how the airplane will fly.
Lift is produced all along the span of the wing, and methods exist to calculate the lift distribution for a given wing. As with the weight acting at the center of gravity, it is common to lump the total lift produced by the wing into a single point known as the center of pressure. The relationship between the center of gravity and the center of pressure is very important when it comes to the controlability and stability of an aircraft.
Lift is a force vector which acts perpendicular to the direction of flight.
Thrust
Thrust is the propulsive force that pushes or pulls an aircraft through the air and is produced by the aircraft’s engines. The resulting thrust force is a function of the position of the engine(s) on the aircraft. There are a few common engine locations as shown below:
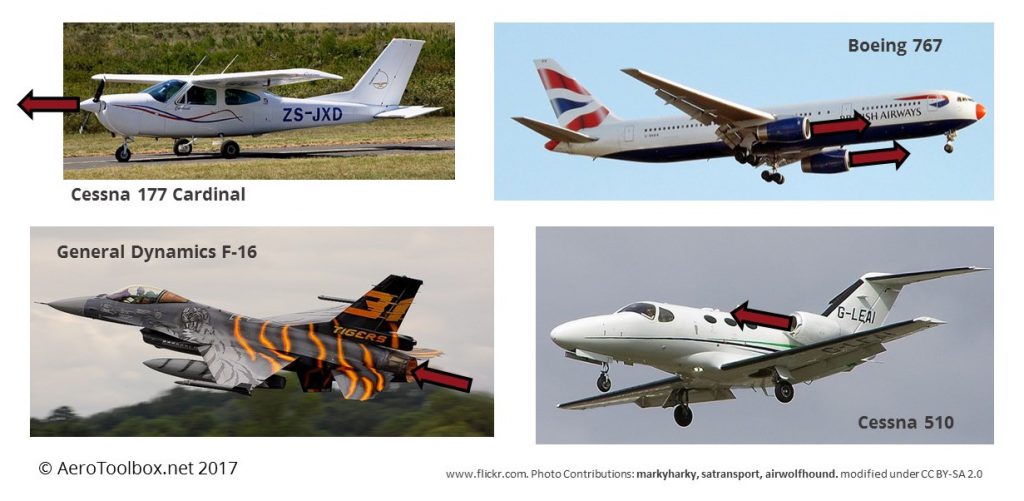
Some common engine locations include: in front of the nose, under the wings, internal to the fuselage, on the rear fuselage.
The thrust generated by an aircraft’s engines is used to overcome the aerodynamic drag associated with moving through the air. In order to fly at a constant speed and altitude, the thrust produced by the engines must be set equal to the total aircraft drag. The total thrust produced is varied using a throttle control which allows an aircraft to accelerate or decelerate depending on the instantaneous difference between thrust and drag.
Remember that while an engine works by pushing air behind itself the reaction force (according to Newton’s third law) generates an equal but opposite force (thrust) which propels the aircraft forward. This is why the thrust direction is forward on all the examples shown above.
Thrust is a force vector which acts in the direction of flight.
Drag
Aerodynamic drag is the force which resists the motion of the aircraft moving through the air. This resistance must be overcome by the thrust of the engines. You can visualize how the air may impede the motion of an aircraft by thinking about how water impedes the motion of a swimmer. The principle is exactly the same although the medium through which the airplane is moving is air rather than water.
We will look at drag in far greater detail as the course progresses; specifically how to account for the total drag as the sum of the individual components of the aircraft, but at this point it is just important to note that the total aircraft drag is proportional to the square of the airplane flying velocity. This means that as the aircraft speed is increased, the drag increases exponentially with the square of speed. Correspondingly, the total thrust required to maintain a constant speed is therefore also a function of the aircraft velocity squared (thrust = drag at constant speed).
Drag is a force vector which opposes the direction of flight.
Putting it all together
So using our four fundamental forces we have the beginning of a model that we can use to predict the motion of an aircraft. The forward motion is largely determined by the thrust and drag. When thrust is greater than drag the aircraft will accelerate in the direction of the motion. The vertical motion is largely determined by the magnitude of the total lift produced by the aircraft (remember that weight is constant when considering a moment in time).
This is clear to see when considering the aircraft in straight and level flight.
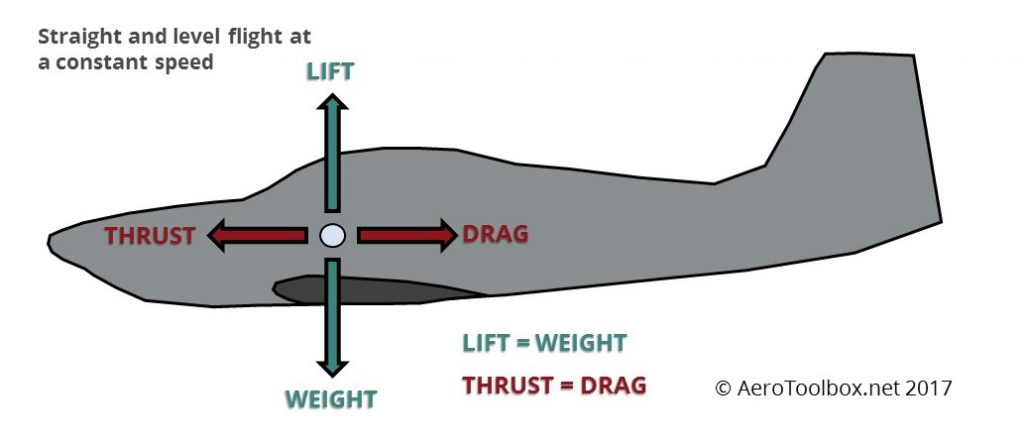
Things get a little more complicated when considering what happens when the aircraft is climbing or descending. Here one needs to use some vector analysis to show how the components of lift and drag interact with the thrust and weight. We have simplified the analysis to assume that the lift and weight act through the same point and that the thrust and drag line are not offset from one another.
From the image below we see that two things happen when an aircraft begins to climb:
- A component of the total weight acts in the same direction as the drag force. This requires that additional thrust be produced to balance the longitudinal forces.
- The total lifting force produced by the wing is LESS than the weight of the aircraft.
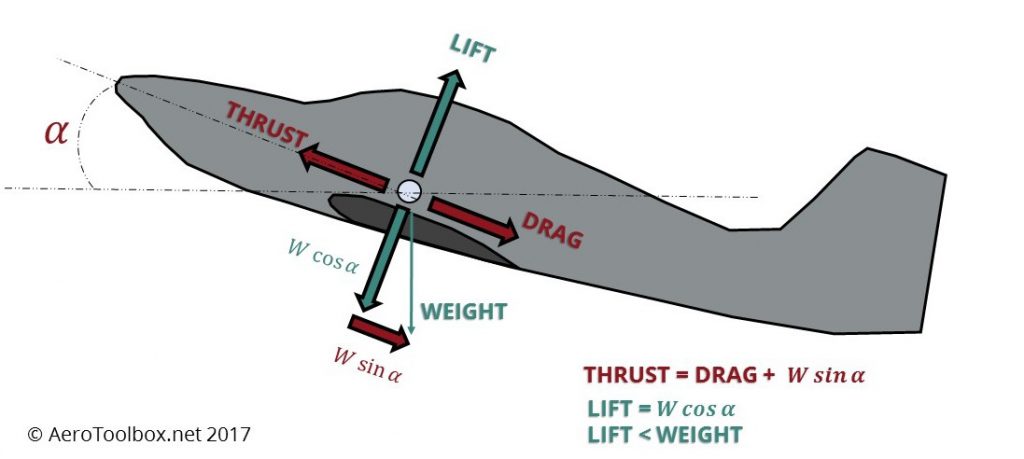
That wraps up this first lesson on the fundamental forces of flight. Next we focus a on the two aerodynamic forces (lift and drag). We will derive three very important non-dimensional qualities; the lift, drag, and pitching moment coefficients which we use to describe the state of an aircraft in flight.
Thanks for reading. If you enjoyed this post and would like to learn more, then hop over to the next installment in the series here.